For a powerful electric vehicle performance, a long-life cycle, higher power density, and an economical battery are required. Li-ion batteries stand out in this regard due to their higher energy and power densities, lengthy life cycles, and widespread use in practically all electric cars. However, lithium-ion batteries have a variety of issues that compromise the effectiveness and safety of electric cars, such as their propensity for overheating and thermal imbalance. The installation of an appropriate battery management system is necessary to get the most out of the electric vehicle.
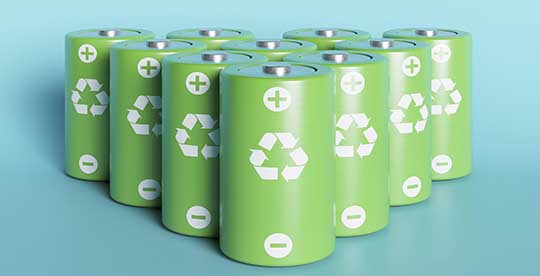
A battery management system (BMS), a piece of technology created to produce a specific range of voltage and current for a length of time against predicted load scenarios, controls a battery pack, an assemblage of battery cells electrically organised in a row by column matrix structure. Undoubtedly challenging, the BMS’s job description may involve aspects of electrical, digital, control, thermal, hydraulic, and other disciplines in addition to being generally complicated. Electric vehicles (EVs), which are now being replaced by internal combustion (IC) engine-based cars, are regarded to be the most advantageous choice. The production of electric cars is based on a major concept that is being developed swiftly in EV technologies: battery technology. How well an EV performs is dependent on the battery’s and the battery management system’s performance (BMS).
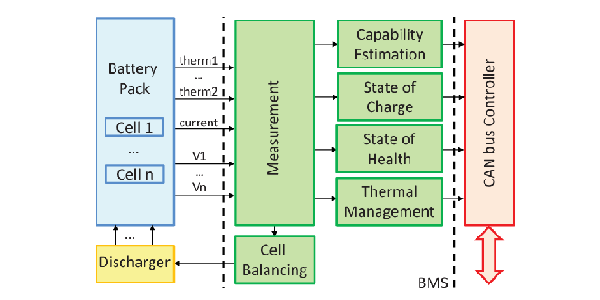
Lithium-ion (Li-ion) batteries are currently predominantly used as batteries in electric cars due to their less weight, better energy density, and rapid charging and discharging capabilities (EVs). The BMS is designed to address issues such battery energy management, heating time reduction at low temperatures, and improved remaining usable life (RUL) with accurate forecast while considering the EVs’ dynamic performance, economics, safety, and environmental friendliness. The BMS oversees the battery’s management and operation, and its main goals are to maintain its reliability and security.
Estimating the battery’s level of charge is essential for vehicle control and energy management. Automobiles that are powered totally or partially by energy are known as electrical cars. Because they have fewer moving parts to maintain, electric cars have lower running costs. They may also be highly environmentally beneficial because they use very little fossil fuel (petrol or diesel). Think about Figure. This EV block diagram shows the battery management system in its simplest form. Through the electronic control unit, the acceleration/pedal is coupled to the power converter. The bidirected electric motor is linked to the power converter. The mechanical drive connects the wheels to the electric motor. The power converter is directly linked to the battery.
The battery and electric control unit are bidirectionally linked to the BMS. The BMS measures and keeps track of the speed at which the vehicle’s batteries are being used for electricity. BMS also keeps an eye on the battery’s temperature, state of charge (SOC), state of health (SOH), overcharging, and over discharging. BMS sends a signal to the electronic control unit when the battery is over discharging. This component generates a control signal to converter and modifies motion speed. Lithium-ion batteries (LIBs), which have high prices, supply risks, production-related resource and energy needs, and environmental problems, have gained attention from a variety of stakeholders with the expanding shift toward electric vehicle fleets. Potential remedies for slowing and closing resource cycles are examined, including circular business models (CBMs) and circular economy (CE) initiatives.
This study evaluates the literature on CE strategies for LIBs and compares their current implementation among European vehicle manufacturers with an emphasis on the components and contributing variables of circular business models. By reviewing company websites and speaking with six company representatives, these were found. Further context-specific and internal characteristics were also discovered through study of a particular firm. Finally, it evaluates the external policy motivations for and constraints of CE methods for LIBs and explores future directions for policy.
The findings show that several firms are implementing CE policies, with a main emphasis on repair, refurbishment, and repurposing. The degree of manufacturer engagement appears to be related to operationalization variation, suggesting that CBMs are context-specific and dependent on internal variables. It was discovered that all CBMs needed tight cooperation amongst many stakeholders to foster confidence and lessen uncertainty. To allow life-extension CBMs, it was emphasised that it was essential to design for disassembly and to have the knowledge necessary to accurately detect the health of LIBs. There is still a need to think about future policy development to maintain adherence to the waste hierarchy, even though the late 2020 plan for new EU law for batteries contains ambitious new standards that would incentivize CE initiatives.
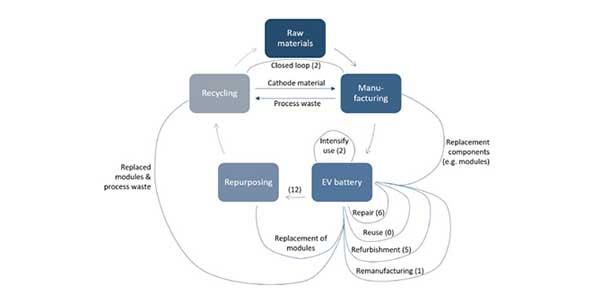
Lewandowski contends that while analysing circular business models, important contextual (internal and external) elements must also be considered for how they affect the application of circular life cycle methods and their operationalization in business models. In this study, external variables are comparable to earlier research for CE strategies for LIBs (cf. Kurdve et al., 2019) and circular business model literature in that they focus on regulatory drives, technology advances, societal pressures, and market considerations. Internal variables have to do with the characteristics that set businesses apart, such as organisational and strategic levels of CBM participation. It was envisaged that the latter would be demonstrated via actions like the development of resources and capacities. This paper analyses internal and external elements that are crucial for this analysis, including the firm strategy, organisational structure, capability, and value networks (cf. 2.4), with a special emphasis on current legislative changes.
How national policy looks in to it
The federal government has announced a variety of promotional steps during the past ten years to aid in the acceptance of electric cars (EVs) in the nation. These policies include tax incentives for EV owners, the building of public EV charging infrastructure, and others.
The mission’s purpose is to advance ideas for revolutionary mobility and phased manufacturing programmes for batteries, components, and electric automobiles. The mission’s major roles, road plan, and estimated impact are as follows:
- Phased Manufacturing Programs for Electric Vehicles, Electric Vehicle Components, and Batteries and Drive Strategies for Transformative Mobility
- Establishing a Phased Manufacturing Program (PMP) to localise manufacturing along the whole value chain for electric vehicles; • Finalizing the localization’s specifics with a defined Make in India approach for both battery and electric vehicle components.
- The Mission will work with important Ministries, Departments, and State stakeholders to coordinate a variety of efforts to alter India’s mobility.
- Phased battery production roadmap with initial emphasis on large-scale module and pack assembly factories by 2019–20 and Gigascale integrated cell manufacture by 2021–22.
- Ensuring holistic and all-encompassing expansion of India’s battery manufacturing sector via PMP
- Improving air quality in cities, lowering India’s reliance on foreign oil imports, and increasing the use of renewable energy and storage technologies are all factors that will have an impact.
- The Mission will establish the plan and strategy that will allow India to take advantage of its size and scale to create a competitive domestic manufacturing sector for electric mobility.
- Provide job possibilities via “Make in India” across a variety of skill sets, with the goal of promoting “Ease of Living” and improving the quality of life for all inhabitants.